Research Interests
The Veterinary and Comparative Neuro-oncology Laboratory (VCNOL) is a translational research group whose primary mission is to develop more-effective methods for the diagnosis and treatment of brain tumors affecting companion animals and humans.
Dr. Rossmeisl's research projects are funded by various federal grant agencies, non-profit organizations, and private sectors, including the National Institutes of Health (NIH), Virginia Biosciences Health Research Corporation (VBHRC), the American Cancer Society, and the Veterinary Memorial Fund at the Virginia-Maryland College of Veterinary Medicine.
Recently, Rossmeisl and his collaborators were awarded prestigious R01 and P01 grants by the NIH, and an overview of the VCNOL's contribution to this ongoing work was published in Glioblastoma.
I: The Canine Spontaneous Brain Tumor Translational Disease Model
Malignant gliomas (MGs) are a group of aggressive and nearly universally fatal brain tumors. Although tremendous investments in MG research have been made over the past several decades, few therapeutics have progressed successfully through Phase III clinical trials. Even with the best available current care, the median survival associated with the most common MG variant in humans, glioblastoma (GBM), is currently less than 2 years.
A significant impediment to the advancement of MG therapeutics remains the lack of faithful preclinical model of MG. Besides humans, dogs are the only known species that spontaneously and frequently develop brain cancer, and gliomas account for approximately one-third of all canine primary brain tumors. Dogs with MG also have a poor prognosis, with death occurring within weeks to months following diagnosis. In both dogs and humans, there is a clear need for brain tumor therapies that improve patient survival and quality of life.
Work done in our laboratory has demonstrated that canine MG share many clinical, radiological (Figure 1), molecular and pathological features with their human counterparts. Dogs with naturally occurring tumors offer additional advantages over studying experimentally-induced cancers in rodents in that they possess an intact immune system, share the same environment as humans, and have brains of sufficient physical size to facilitate the testing and optimization of both diagnostics and therapeutics developed for human patients without a need to rescale instrumentation. Our goal is to improve the lives of canine patients with brain cancer, and use the canine spontaneous brain tumor model to develop novel therapeutics for humans. This goal is achieved primarily through canine brain tumor clinical trials.
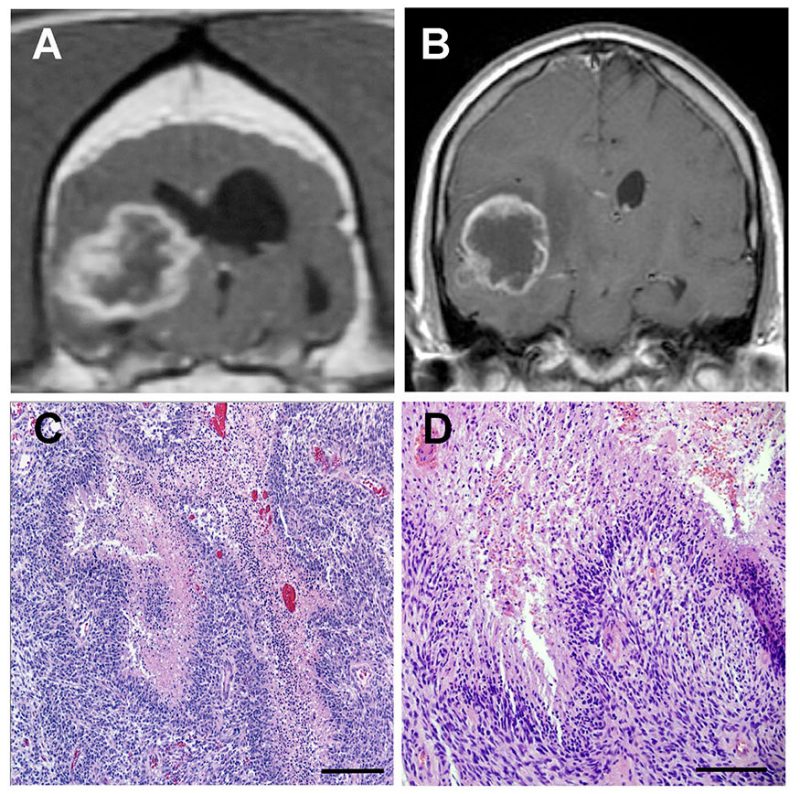
II: Precision Medicine for Brain Tumors: Molecularly Targeted Therapeutics (MTT)
Many of the conventional chemotherapeutic drugs used clinical practice are relatively non-specific with regards to their cellular “targets.” Essentially, these agents will kill or otherwise alter all cells that are exposed to adequate drug concentrations. The clinical consequences of this non-specific action is that most chemotherapeutic agents result in damage to or death of normal, healthy cells in addition to cancerous cells. It is these effects on healthy cells that give rise to the notorious and systemic adverse effects associated with traditional chemotherapeutics. Modern cancer research has been heavily devoted to the development of MTT, drugs that are specifically designed to trigger cell death by “targeting” unique aspects of cancer cells, such as cell surface proteins, that differentiate them from normal cells. Some MTT, such as tyrosine kinase inhibitors, are already in use in human and veterinary clinical practice and have shown promise in the treatment of a number of cancers. MTT are important components of tumor immunotherapy and precision medicine.
In conjunction with the molecular therapeutics laboratory directed by Waldemar Debinski, we have identified and generated novel cytotoxic agents that target two cell surface proteins, IL-13Rα2 and EphA2, that are expressed on the majority of canine gliomas but are not present in normal canine brain tissue (Figure 2). These bacterial cytotoxins are potent and specific MTT against brain cancer cells and spare normal brain tissue. We are currently conducting a clinical trial in dogs with malignant gliomas to evaluate this MTT.
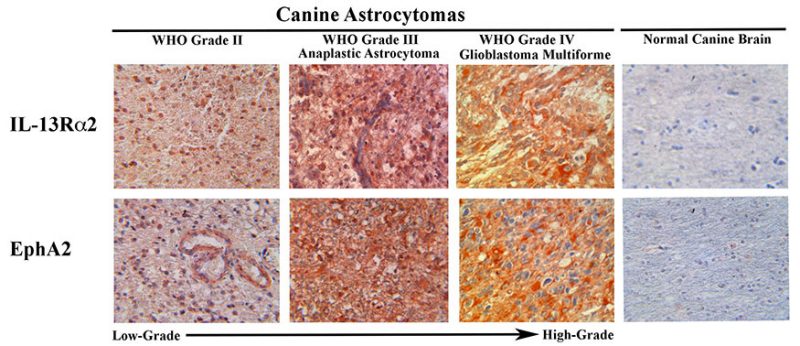
III: Minimally Invasive Cellular Ablative Techniques
In collaboration with Rafael Davalos, the inventor of irreversible electroporation (IRE), we have pioneered the use of IRE for the treatment of brain tumors. IRE is a new and minimally invasive surgical technique to treat cancer. IRE destroys cancerous tissue by exposing cells to a series of short and intense electric pulses via small electrodes placed into or around the tumor. The applied electrical pulses result in the formation of permanent nanopores in cell membranes, which increases the permeability of the cells, and eventually leads to their death without the need for potentially toxic chemotherapeutic drugs. Electroporation can be imaged (Figure 3) and controlled in real time using electrical impedence tomography, which allows the treating clinician to actively monitor the procedure.
We have completed clinical trials in dogs in which we have demonstrated the safety and feasibility of using IRE to treat brain cancer, and also provided preliminary data with respect to the effectiveness of IRE for the ablation of gliomas. Our current studies are investigating the use new generation electroporation platform, capable of delivering high-frequency irreversible electroporation (HFIRE) pulses for the ablation of brain tumors. We have successfully treated several dogs with brain tumors using HFIRE (Figure 4).
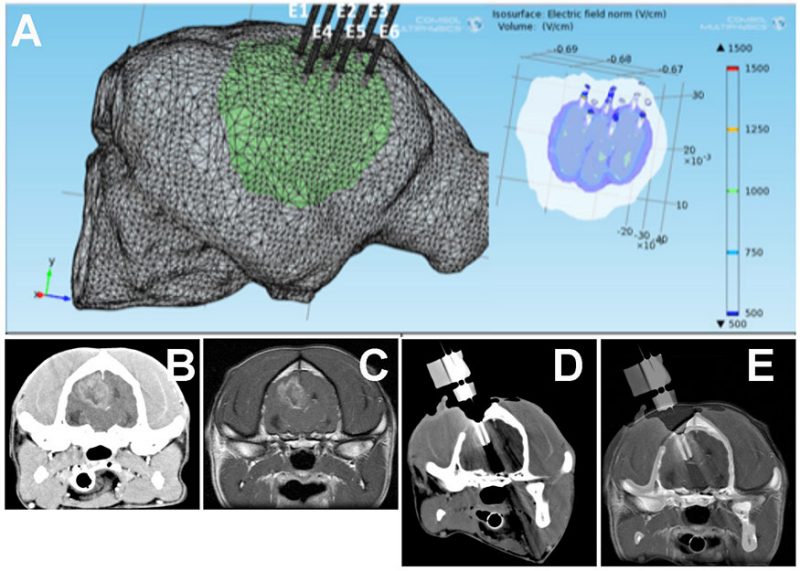
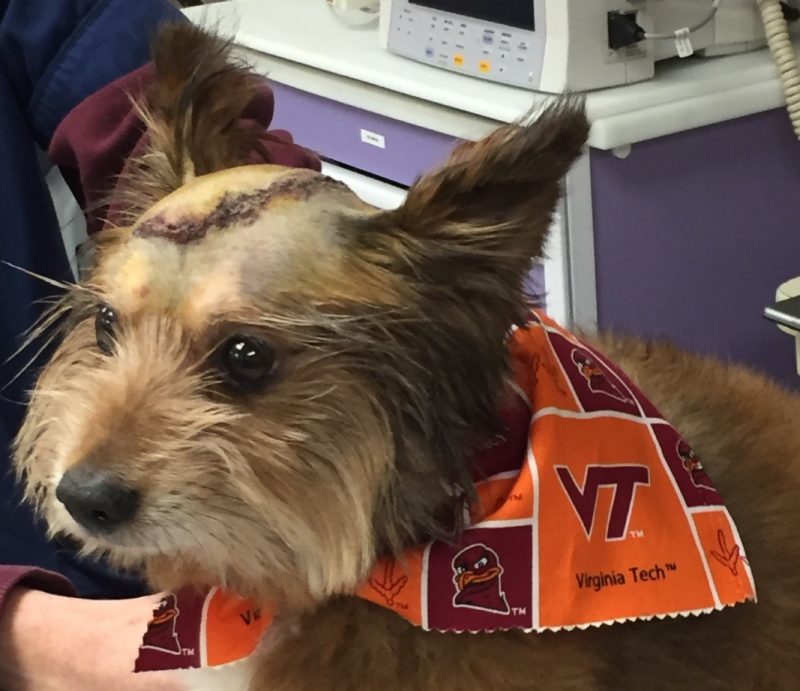
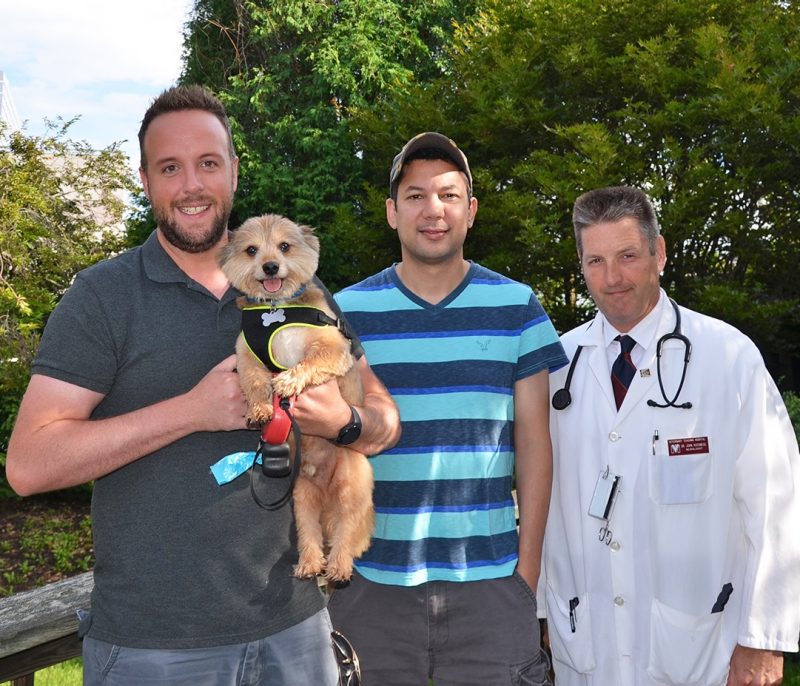
IV: Novel Systems for Drug Delivery to the Brain
One of the major obstacles to successful brain tumor treatments is associated with the impermeability of blood-brain-barrier (BBB) to the vast majority of therapeutic agents. Chemotherapeutic drugs that are administered orally or by systemic injection penetrate brain tissue poorly, which often results in the development of dose-limiting toxicities prior to achievement of therapeutic drug concentrations within the tumor. Accordingly, a significant body of brain tumor research has been devoted to the development of agents and methods that can overcome the limitations imposed by the BBB.
Blood-Brain-Barrier Bypass
Convection Enhanced Delivery (CED) is a therapeutic strategy that was developed to facilitate targeted delivery of pharmaceuticals into the brain. CED is one of the major therapeutic delivery platforms used in our brain tumor clinical trials. The CED procedure involves a minimally invasive surgical exposure of the brain, followed by placement of small diameter catheters directly into the brain tumor. Infusion of therapeutics through the catheters into the tumor over several hours enables saturation of large volumes of target tissue. As this approach effectively bypasses the BBB, it allows for delivery macromolecular drugs that would not normally enter the brain to effectively reach high concentrations within brain tumor tissue.
Building on the experience of other investigators, we perform CED procedures using MRI guidance (Video 1) by coinfusing therapeutic drugs and MRI contrast agents, which allows confirmation of catheter placement, document satisfactory infusion of the targeted region, and monitoring the patient for the development of complications. In partnership with Chris Rylander’s laboratory, we have also developed novel catheter systems to perform CED more precisely and efficiently. Collectively, our technical advancements are designed break down the barriers that have historically been associated with the CED procedure. Our newest generation CED platform is a fully integrated, computer controlled, and image-guided system that will allow neurosurgeons to deliver therapeutic agents into any part of the brain.
Blood-Brain-Barrier Disruption
We have discovered that IRE and HFIRE pulses are capable of transiently disrupting the BBB in a region of brain tissue surrounding the zone of ablation in a voltage-dependent manner. We have also demonstrated that through the alteration of pulse parameters such that the electrical energy delivered to cells is sublethal, IRE and HFIRE pulses can selectively open the BBB. The mechanism by which electroporation-induced BBB disruption occurs is unknown, and is a focus of our current research. Thus, electroporation-based therapies offer distinct advantages for use in brain cancer treatment. With a single treatment, IRE and HFIRE are capable of both the direct killing of cancer cells and the simultaneous opening of the BBB, which could be exploited to deliver additional anti-cancer agents to the brain tumor.
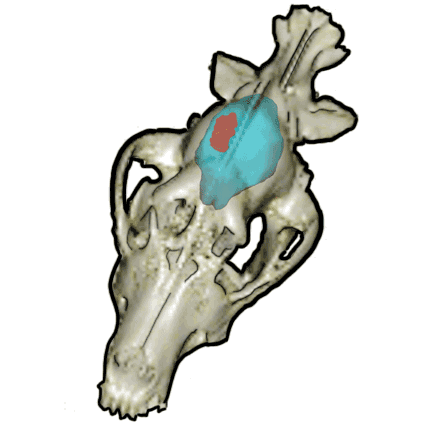
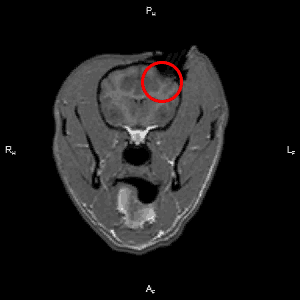